What Happens In All Energy Transformations?
An energy transformation refers to the change of energy from one form to another. Energy can take many different forms, such as kinetic energy, potential energy, thermal energy, chemical energy, nuclear energy, electrical energy, and more. Energy transformations occur constantly around us, from photosynthesis in plants to electricity generation in power plants.
Understanding energy transformations is crucial because we rely on them for almost every human activity and modern convenience. For example, a car engine transforms the chemical energy stored in gasoline into kinetic energy that powers the wheels. In a solar panel, radiant light energy from the sun gets transformed into electrical energy that can power devices and appliances. Even living organisms like humans constantly transform chemical energy from food into kinetic energy to move around and thermal energy to maintain body temperature.
Studying energy transformations allows us to better harness and control energy for human needs. It also sheds light on the limits and inefficiencies inherent to any energy transformation process. With a deeper knowledge of how energy transformations work, we can develop new technologies and improve existing processes to use energy more efficiently and sustainably.
Laws of Thermodynamics
The laws of thermodynamics describe the relationships between thermal energy, mechanical work, and energy transformations. There are three main laws of thermodynamics:
The first law states that energy can be converted from one form to another, but it cannot be created or destroyed. This is known as the law of conservation of energy. The total energy in a closed system remains constant. For example, when an object falls, its potential energy is converted to kinetic energy.
The second law states that in any closed system, the entropy (disorder) increases over time. Entropy is a measure of randomness or disorder in a system. As energy transformations occur, some energy escapes or gets dispersed as heat, increasing the disorder of the system. For example, heat always flows from hot to cold objects.
The third law states that entropy approaches a minimum value as the temperature of a system approaches absolute zero. Absolute zero is the lowest theoretically possible temperature, at which a system would have no thermal energy. The third law helps define absolute scales for entropy and temperature.
Together, these laws describe how energy moves and changes in a closed system. The laws of thermodynamics have profound effects on natural processes and technology.
Forms of Energy
There are different forms that energy can take. Some of the main forms of energy include:
Potential Energy – This is energy that is stored due to an object’s position or configuration. For example, a ball held at a height has potential energy due to gravity. When released, this potential energy gets converted to kinetic energy as the ball falls.
Kinetic Energy – This is the energy possessed by an object due to its motion. The faster an object moves, the more kinetic energy it possesses.
Thermal Energy – Also known as heat, this is the energy transferred between objects or systems due to a temperature difference. Thermal energy flows from higher temperature to lower temperature objects.
Chemical Energy – This is the potential energy stored in the bonds between atoms and molecules. Chemical energy can be released during chemical reactions.
Nuclear Energy – This energy comes from the splitting (fission) or joining (fusion) of atomic nuclei. Nuclear power plants split uranium atoms to release energy.
Electrical Energy – This is the energy from the flow of electrons. It is common in technology, such as in batteries, generators, and electrical circuits.
Radiant Energy – This is energy transported by electromagnetic waves, such as light, radio waves, microwaves, and X-rays. Radiant energy can propagate through space.
Energy Transformations in Nature
Energy transformations are constantly occurring in the natural world. Some of the most important energy transformations take place through biological processes like photosynthesis and cellular respiration.
During photosynthesis, plants use energy from sunlight to convert carbon dioxide and water into glucose (food) and oxygen. This process stores energy from light in the chemical bonds of glucose molecules. The glucose can then be broken down through cellular respiration to release energy that fuels plant growth and metabolism.
Photosynthesis and cellular respiration make up an energy cycle that powers life on Earth. Plants convert light energy into chemical energy that gets passed through food chains. Herbivores eat plants, carnivores eat herbivores, and so on. At each stage, cells break down glucose and convert the stored chemical energy into forms that organisms can use to move, grow, and reproduce.
In summary, photosynthesis and cellular respiration allow energy from the Sun to be harnessed and transformed to support life. These biological energy transformations are essential processes that sustain food webs and natural ecosystems.
Energy Transformations in Technology
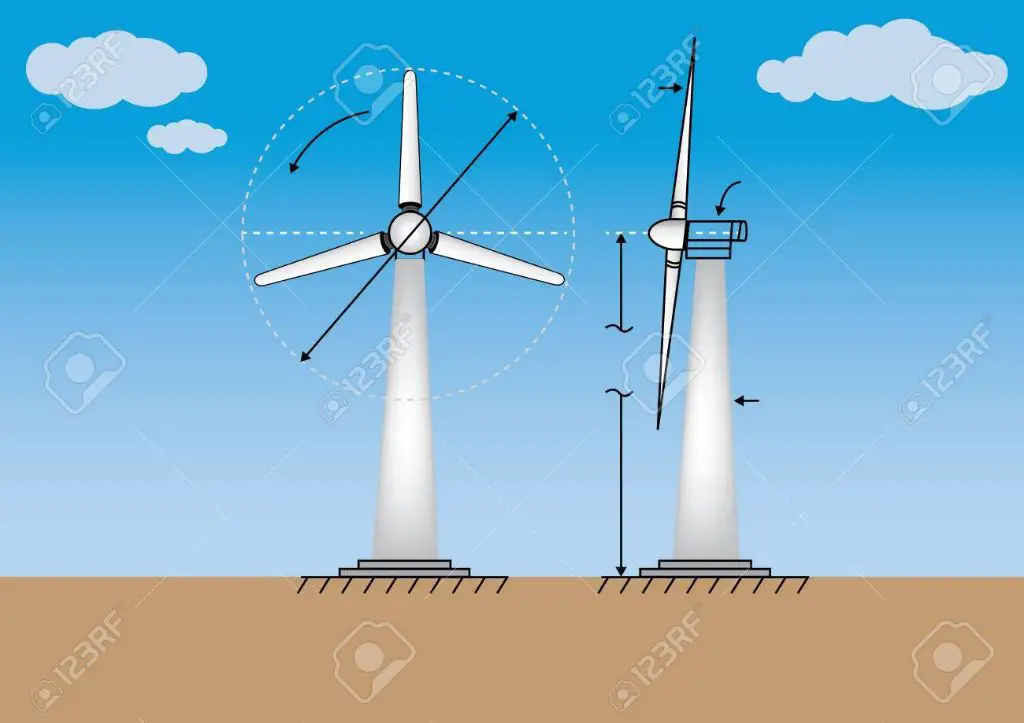
Technology relies on the transformation of energy to perform useful work. Some key examples of energy transformations in technology include:
Combustion Engines: In combustion engines like those found in cars and trucks, the chemical energy stored in fuel is transformed into thermal energy through combustion. This thermal energy creates pressure that pushes pistons, transforming the thermal energy into mechanical energy that powers the vehicle.
Batteries: Batteries transform chemical energy into electrical energy through redox reactions. The structure of the battery regulates the reaction rate to provide a steady electric current that can power various devices. Rechargeable batteries can reverse the reaction by applying an external electrical current to restore the battery’s chemical composition.
Power Plants: Power plants operate on various principles to transform energy for electricity generation. Coal and natural gas plants burn fuel to boil water into steam that drives turbine generators. Nuclear plants use nuclear fission to create heat and steam. Hydroelectric plants convert the gravitational potential energy of falling water into electricity via turbine generators.
Understanding these energy transformations enables engineers to design technology to efficiently convert energy into useful forms. Mastering energy conversions allows society to harness power sources like fossil fuels, nuclear reactions, and renewable sources.
Conservation of Mass-Energy
One of the most revolutionary concepts in physics is the principle of mass-energy equivalence. This states that matter can be converted into energy, and energy can be converted into matter, according to Einstein’s famous equation E=mc^2.
This means that mass (m) and energy (E) are interchangeable and can be expressed in terms of one another. The speed of light (c) is the conversion factor. Even a tiny amount of matter contains an enormous amount of energy due to the giant magnitude of the speed of light squared.
For example, the mass loss in nuclear reactions gets converted to energy as per E=mc^2. This could involve matter splitting apart into smaller nuclei (fission) or matter combining to form larger nuclei (fusion). The ‘lost’ mass gets converted into the kinetic energy of the reaction products.
This conservation of total mass-energy is a fundamental principle of physics. The total amount of mass and energy before and after any process remains constant. But thanks to E=mc^2, we now know that mass and energy are interchangeable forms of the same underlying substance.
Efficiency
Efficiency measures the ratio of useful output energy to the total energy input in any energy transformation. It provides a quantitative basis for comparing how well different energy transformations convert the available energy into usable work.
Efficiency is expressed as a percentage or decimal between 0 and 1 (or 0 and 100%). An efficiency of 100% would represent a perfect energy transformation with no energy wasted. However, in real world systems, some energy is always lost to heat, friction, or other unintended forms.
For example, a lightbulb may transform only 10% of the electrical input energy into visible light energy, wasting the rest as heat. An electric motor may operate at over 90% efficiency in transforming electrical energy into rotational kinetic energy. Fuel cells can utilize 40-60% of the available chemical energy in hydrogen.
Improving energy efficiency is an important strategy for reducing energy waste. Technologies can often be designed to maximize desired energy outputs while minimizing unusable byproducts. However, thermodynamic limits and economic factors place practical constraints on how efficient any system can be in real-world applications.
Understanding efficiency helps identify the most effective energy sources and transformations for particular human needs. Comparing input to output energies highlights relative advantages among competing technologies or processes. Efficiency also provides key insight into energy conservation opportunities and the long-term sustainability of energy use.
Heat and Work
One of the most common energy transformations is the conversion of heat into mechanical work. This occurs in heat engines, where thermal energy from a high-temperature heat source is converted into useful mechanical work.
For example, in a steam engine, the heat from a boiler converts water into high-pressure steam. This steam then pushes a piston to turn a crankshaft and produce rotational kinetic energy. The steam’s thermal energy is transformed into the mechanical energy of the moving piston and crankshaft.
Similar heat engines include internal combustion engines in cars and gas turbines in jet engines. In these systems, heat from the combustion of fuel generates expanding gases that drive the motion of the engine. The thermal energy is changed into mechanical energy that propels the vehicle.
On a molecular level, heat represents the random kinetic energy of molecules. As the molecules collide with the walls of an engine, their momentum is transferred to the larger mechanical system, organized into the motion of the pistons, wheels, etc. The higher the temperature, the faster the molecular motion, which enables more work to be performed.
In all these examples of motors and engines, heat and thermal energy are transformed into mechanical work. This is a key energy transformation that powers much of today’s transportation technology through the generation of useful work from thermal energy.
Real-World Applications
Energy transformations occur constantly in the real world, powering many aspects of our daily lives. Here are some examples of energy transformations in action:
Energy Production: Fossil fuels like coal, oil, and natural gas undergo combustion reactions to produce heat energy that is used to boil water into steam that spins turbines to generate electricity. Nuclear power plants split uranium atoms to release energy that heats water into steam that drives electricity-generating turbines. Renewable sources like solar panels convert sunlight into electrical energy.
Transportation: Gasoline and diesel fuels are ignited in car and truck engines, undergoing combustion reactions that release energy to move pistons that turn a crankshaft, thus moving the wheels. Hybrid and electric vehicles use electrical energy stored in batteries to power electric motors that turn the wheels.
Electronics: Batteries in devices like cellphones and laptops transform chemical energy into electrical energy that powers the device. Chargers transform electrical outlet energy back into chemical energy stored in the battery.
Conclusion
As we’ve seen, the laws of thermodynamics govern all energy transformations that occur in nature and in human technology. The first law states that energy can neither be created nor destroyed – it can only change form. This means that the total energy in a closed system always remains constant.
The second law states that in any energy transformation, some energy is always lost to heat. This lost energy can never be fully recovered, and this drives all physical processes in one direction only. While 100% efficiency is impossible, we can strive to maximize efficiency in energy transformations and minimize unnecessary waste and heat loss.
Understanding these fundamental laws allows us to better grasp natural processes like photosynthesis, digestion, and weather systems. It also informs the design and improvement of human technologies like engines, power plants, and batteries. The study of thermodynamics has enabled incredible advances in engineering and industry.
Energy lies at the heart of all activity in the universe. The orderly transformation of energy from one form to another makes all motion, life, and intelligence possible. Conserving energy and using it wisely are critical to protecting our planet and securing our future.